Canada is a large country with vast distances between remote communities and national grids. There is a shortage of long-distance electricity transmission lines, so these remote communities have had to rely on Distributed Power Generation which was historically Coal, Heavy Fuel Oil (HFO), or Diesel powered. With the Energy Transition, these remote communities are considering their Renewable power options. Hybrid Microgrids are an attractive option to increase the use of Renewables whilst maintaining grid stability and reliability.
Canada is the second largest country in the World. It is a diverse country with a range of climates, economies, and living standards. It is 10 million square kilometres; larger than the US and twice the size of Europe. The distance from North to South is ~4,600 kilometres. From East to West, the distance is ~5,800 kilometres.

The Köppen Climate Map illustrates climatic regions with varying amounts of cloud cover, precipitation and wind energy distribution:

Canada is roughly divided in half – the southern half gets more solar radiation, whilst the northern half is affected by shorter days in the winter, reducing annual solar radiation. Fortunately, the northern half generally gets more wind energy. Western Canada has less solar radiation due to more precipitation and has widely varying wind energy due to rugged terrain. There does appear to be technical solutions to increase Renewable power generation.
Solar
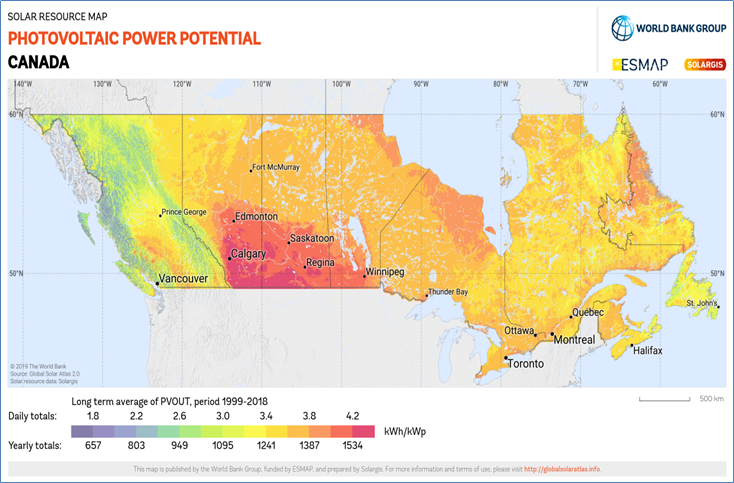
Wind
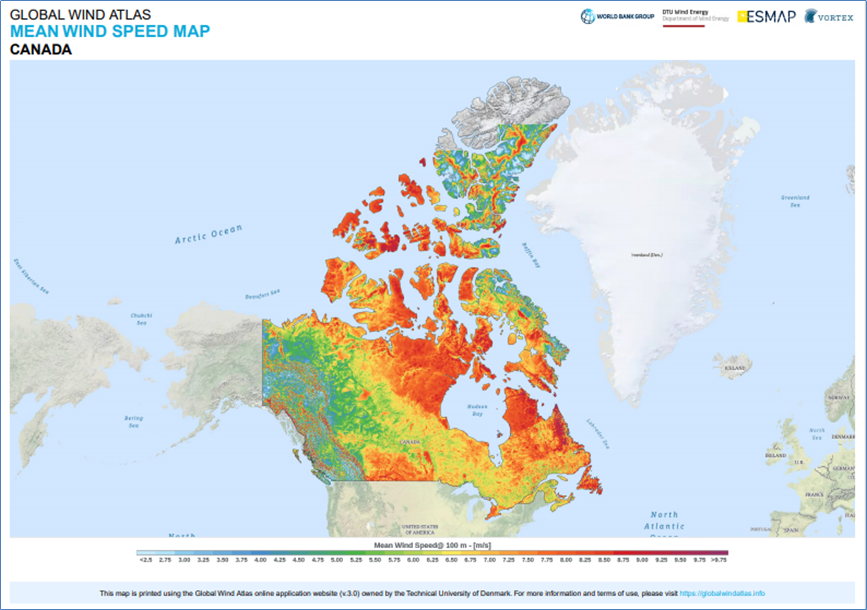
Remote Communities in Canada
It has been estimated that Canada had 279 active remote communities that were not connected to the North American electric grid. About 239 of these communities relied on diesel fuel for electricity and heavy fuel oil (HFO) for heating. Two thirds of these communities were Indigenous. [3] It has also been estimated that 25-50% of these Indigenous communities have electrical load restrictions due to inadequate and aging Conventional Power Generation equipment. Load restrictions mean constraints on further economic development, including housing, commercial establishments, education, and improved sanitation facilities. The Diesel-based electrical power uses ~195 million litres of fuel per year. Fuel oil-based heating uses another ~116 million litres of fuel per year. Unfortunately, some of this liquid fuel has been spilled, resulting in ~1400 contaminated sites. The cost of Diesel and Fuel oil is significantly increased with the logistical challenges of year-round transport and long-term storage in these remote areas. In addition to reducing these high costs, mitigating GHG Emissions and avoiding potential groundwater pollution are good reasons for increased deployment of Renewables.


Renewable Power for Remote Communities
The preceding maps of Solar radiation (Solargis) and Wind energy (Global Wind Atlas) show that Canada is able to be roughly split into northern and southern regions with different amounts of Solar radiation and ranges of Mean Wind Speeds.
From experience, the intermittency of these Renewables means that hybrid solutions with both types, combined with Energy Storage Systems (e.g. Lithium-ion or Lithium Iron Phosphate (LFP) batteries for short duration storage and grid stability) may be the best solution. With seasonal climate patterns (e.g. long winter nights and snow), long-duration storage would also likely be applicable (e.g. Hydrogen P2G2P).
A review of Solargis’ Photovoltaic Electricity Potential (PEP) maps gives relevant Solar Power data[4]. Solargris’ maps provide long-term averages of daily/yearly potential electricity production from a 1 kW Solar PV power plant. The assumed PV system configuration consisted of ground-based, free-standing structures, with crystalline-silicon PV modules mounted at a fixed position with optimum tilt to maximize yearly energy yield. The use of high efficiency inverters is assumed in their maps. An alternate source for PEP is Natural Resources Canada.[5]
A review of Global Wind Atlas wind maps gives some relevant Wind Power data[6]. Global Wind Atlas’ maps provide Mean Wind Speeds able to be used with a typical Wind Turbine Power Curve – in this example it was assumed to be the GE 1.7-100 Wind Turbine. The assumed number of Wind Turbines can be calculated from this information.
For an assumed medium sized remote community with residential, some businesses, and small industrial users, the assumed power demand requirement would vary over the course of the 24 hours. For this example, the nominal demand and supply targets could be as follows (below left). If Solar Power contribution is not feasible, more Wind Power generation would be needed (below right). Depending on Wind intermittency, ESS may be required other than for grid stability.
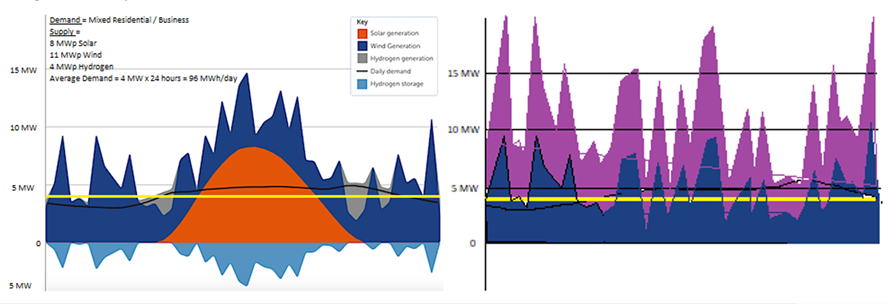
- Peak demand ~5 MWp; Average demand ~4 MW x 24 hours x 365 days = 35,040 MWh = 35,040,000 kWh total power needed= MWhreqd (split in some percentage between Solar and Wind);
- Solar:
- Mixed Solar Scenario: assume Solar 8 MWp required x 24 hours x 365 days = MWhSolar (day time);
- Assume 1 MWp plant requires 2632# x 380 W Solar PV panels; and 1 hectare for 1 MW;
- Cost of PV panels ranges, but $0.37/W for a 380 W panel delivered is conservative;
- Cost of Solar PV panels is assumed to be ~1/2 of the Gross Cost of a Solar PV Farm (on this scale);
- Wind:
- Mixed Wind Scenario: calculate MWp required (from MWhreqd-MWhSolar, all day and night);
- Wind Only Scenario: calculate MWp required (from MWhreqd, all day and night);
- Assuming the required number of turbines is MWp/(Turbine Applicable MWp @ Mean Wind Speed);
- Mean Wind Speed for this example to be assumed to correspond to “50% of windiest areas”;
- Wind Farm Size varies according to land topography and access constraints, but an average value of 1 hectare / Wind Turbine has been suggested;
- Market cost of Wind Turbines have been changing rapidly, but $2MM for a GE-1.7-100 Wind Turbine delivered is used (~$1.1MM/MW);
- Delivered Cost of Wind Turbines are assumed to be ~1/2 of the Gross Cost of a Wind Farm;
From the preceding assumptions and data:
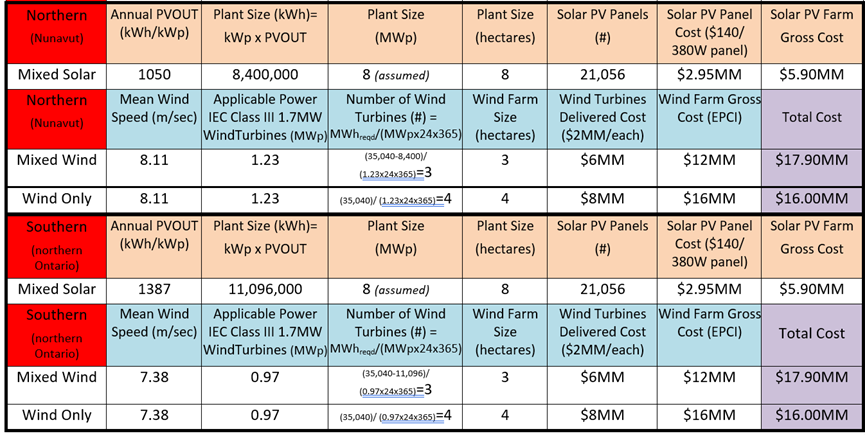
Canada has good wind energy distribution, but local topography and wind data could also be investigated to see if mountain or hill ridgelines are available for siting Wind Turbines in areas of even higher wind speeds. Wind Turbines have been used in all regions of Canada. Wind Power does seem to be the most important energy solution for these regions, given the challenges of seasonal Solar intermittency. It is likely that both would have to be installed to improve resilience.
Added to these costs may be the cost of a high capacity, long-duration Energy Storage System (e.g. Hydrogen P2G2P). It is also possible that a hybrid power generation system for some locations could include small amounts of Conventional Power Generation (e.g. road transported CNG, LNG, or LPG Gas fired engine power generators).
Diesel or Gas fired engine power generators cost ~$1-2MM/MW (~$5-10MM for this 5 MW example). Conventional Power Generation would have OPEX costs including fuel, increased maintenance costs for the rotating equipment, and increased numbers of operations and maintenance personnel. Also not considered in this cost comparison is any Regulatory costs associated with the GHG emissions of Conventional Power Generation. ESG considerations may be part of the decision to adopt hybrid Renewable solutions, in order to improve access to funding and finance.

Role of Clean Gas Power Generation in Canada’s Energy Transition
As you can see in the preceding sections, Solar and Wind Renewables may be challenged in some parts of Canada due to unfavourable prevailing climate conditions and current costs of the equipment. Other types of Renewables may be applicable in the future with more technological advancement and development work. In the meantime, Clean Gas Power Generation could have an important role in the Energy Transition from other more carbon intensive fuels like Coal, Heavy Fuel Oil (HFO) and Diesel. Clean Gas Power Generation emits fewer GHG and other pollutants and it has been characterised as a “bridge” in the Energy Transition and a key part of the “energy mix”.[7] Political and market challenges however remain in both countries.
Canada is one of the best users of Renewables, but it is mainly Hydroelectric Power[8] and the National Grid only covers the southern, more populated areas. Canada is an energy intensive country, partly due to the colder climate and corresponding heating requirements. Off-grid communities have historically used Distributed Conventional Power Generation, either Coal, HFO, or Diesel. The transport of liquid fuels may be able to be replaced by Clean Gas. Without a substantial LNG export capability in Canada, there may be market opportunities for more domestic Gas usage to help progress the Energy Transition here.
Canada has good reserves of Natural Gas across the country, so it should be available in some form. Clean Gas can mean Methane in some form (i.e. Pipeline Gas, Compressed Natural Gas (CNG), Liquified Natural Gas (LNG)), or Liquified Petroleum Gas (LPG) including Butane and Propane. Depending on the type of end user and the distance from Gas reservoir source (or processing plant) to market, each type of Clean Gas will likely find a good application across these countries. A shortage of long-distance Gas transmission pipelines and Electricity Distribution transmission lines to remote northern communities means that some local power solutions would be relevant. Hybrid Microgrids are a good solution to combine Clean Gas Power Generation with Renewables. The Clean Gas component can mean road transported CNG, LNG, or LPG to Distributed Power Generation plants. These plants would be linked, as shown below, to the Renewables in Hybrid Microgrids to support Industrial, Commercial, and Residential users. Investors need to support these hybrid solutions to deliver better environmental, health, and developmental outcomes, particularly for remote Indigenous communities in Canada.


[1] https://solargis.com/maps-and-gis-data/download/canada
[2] https://globalwindatlas.info/en/area/Canada?print=true
[3] http://wgsi.org/sites/wgsi-live.pi.local/files/Energy_Access_Canadian_Context_Infographic_Spread-OpenAccess_Energy_Blueprint_WGSI_2017.pdf
[4] https://solargis.com/maps-and-gis-data/download/africa
[5] https://www.nrcan.gc.ca/sites/www.nrcan.gc.ca/files/canmetenergy/files/pubs/2006-046_OP-J_411-SOLRES_PV+map.pdf
[6] https://globalwindatlas.info/
[7] https://www.atlanticcouncil.org/blogs/new-atlanticist/gas-in-the-energy-transition-bridge-or-the-destination/